Fundamentals of electricity markets
This chapter provides a basic understanding of the fundamentals of electricity wholesale markets. This is done by outlining the scope, describing the demand and supply intersection, explaining market coupling, covering relevant technologies, delimit dispatch from investment, and introducing frequently used units.
Scope
Electricity markets form key elements in the value chain for electricity as shown in the following figure.

The value chain of the electric power industry begins with the development of new technologies for generating, transporting, distributing and consuming electricity. Economically viable technologies drive new infrastructure investments, leading to the construction and deconstruction of generators, grid elements and consumption facilities. This initial stage establishes the generation, transmission, distribution and consumption capacities available. The next part of the value chain can be divided into markets and grids.
Open wholesale markets facilitate the buying and selling of bulk electricity volumes to derive commercial schedules of facilities. These markets coordinate the activities of market participants, grid operators, and their physical assets to optimize the system for maximum total social welfare. In the following retail market, electricity is resold in smaller volumes at higher prices due to added taxes, levies and fees.
In parallel to the markets, transmission and distribution grids ensure the physical transmission and distribution of electricity. Grid operators hold territorial monopolies since constructing multiple grids is not viable, so grid operators are regulated by agencies. These grid operators fulfill the market outcomes in form of commercial schedules through enabling the physical flows of electricity from generators to consumers.
The final stage of the value chain involves using electricity to produce or consume other goods and services.
Maon focuses on simulating electricity wholesale markets with three primary outputs. The first is the short-term equilibrium, where generation, grid, and consumption capacities are inputs used to derive dispatch schedules and wholesale prices. The second output is the long-term equilibrium, which describes investments in generation, grid, and consumption facilities based on projected wholesale prices, volumes, and emerging technologies. The third output quantifies uncertainties in both equilibria and derived results, including revenues and costs for individual facilities.
Demand and supply
The core market principle is to determine prices and volumes through the demand and supply equilibrium, as shown in the figure below.

The equilibrium between demand and supply in each bidding zone determines the wholesale price. On the supply side, the merit order represents the ranking of available generators by ascending variable cost, setting the order in which they are used. Demand consists of both price-inelastic consumers, who are willing to pay any price, and price-elastic consumers, who adjust their consumption based on price changes. Generators with the lowest costs supply each additional required MWh until demand is met, with the last (and highest-cost) accepted bid setting the price and volume. This market-clearing price applies to all participants within the bidding zone.
Demand and supply curves represent the aggregated bids of market participants and their specific technologies used for generation, consumption, and exchange. This aggregation ensures that the market-clearing volume includes the schedule for each component (unit commitment).
Imports and exports between bidding zones, managed through market coupling, are also incorporated into demand and supply (market coupling).
Market coupling
Exchanges via interconnectors allow demand and supply to be shared across the connected bidding zones, as illustrated in the figure below.

Exchanges represent commercial flows, typically moving electricity from lower-priced to higher-priced bidding zones, improving price convergence until prices equalize. When the interconnector capacity is sufficently large, prices equalize. If the capacity is insufficient, a price difference remains, as shown in the figure.
Interconnector capacities are determined by various capacity allocation methods in the market, such as net transfer capacities (NTC), coordinated net transfer capacities (CNTC), and flow-based market coupling (FBMC). These allocations create significant price and volume interdependencies between bidding zones and enhance total social welfare in the electricity wholesale market.
Technologies
The hourly energy balance through technologies for generation and consumption can be seen be seen in the following figure.
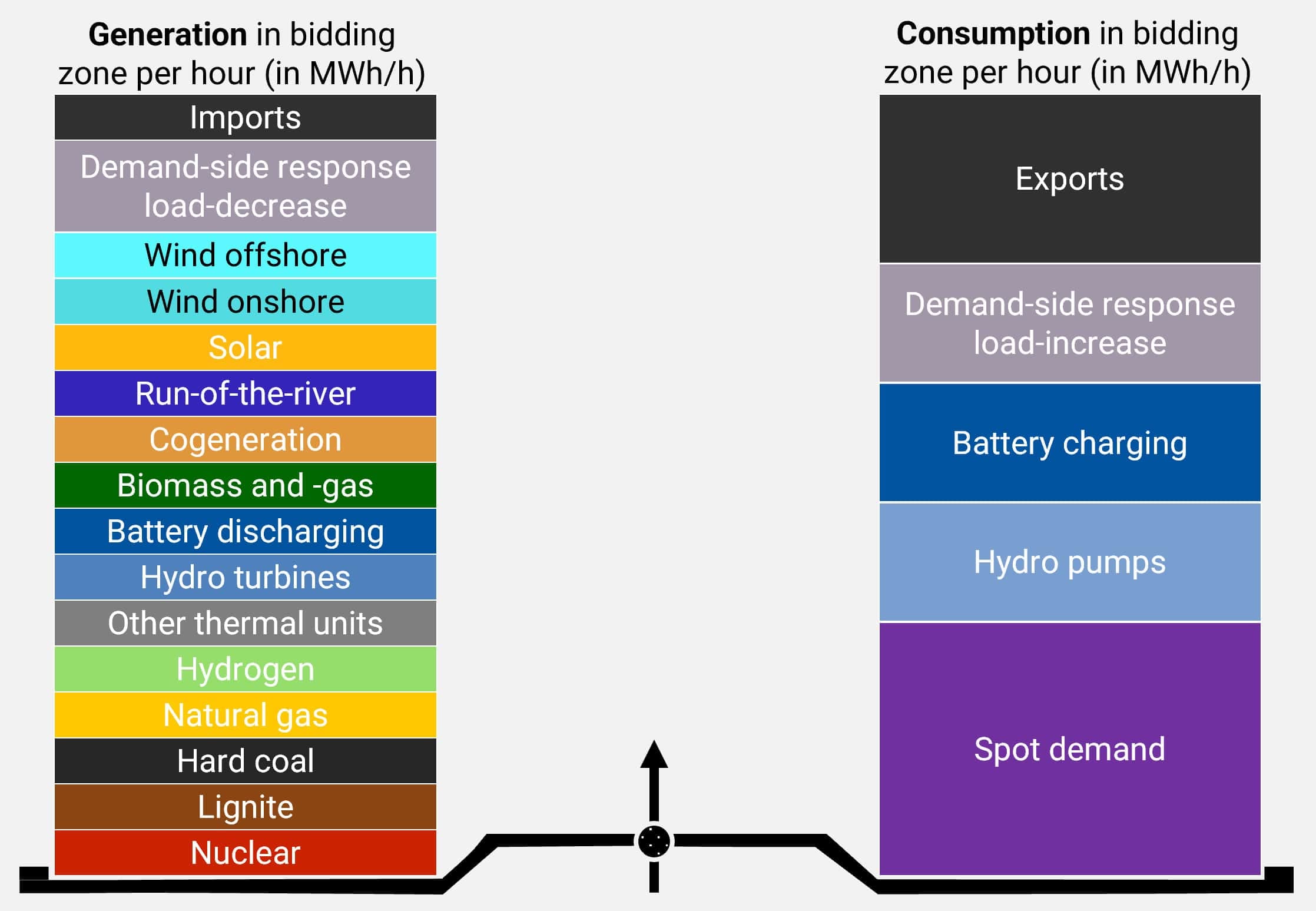
Due to the conservation of energy, electricity generation must match consumption at every moment. This balance also applies on a hourly or quarter-hourly basis within each bidding zone and can be represented in the market as an equilibrium equation. When the price-inelastic demand cannot be met within this equation, the resulting deviation leads to either a surplus (dump energy) or a deficit (energy not served). The equilibrium in the market is collectively established by all market participants and their facilities, taking into account their unique technological and operational flexibilities.
Inflexible consumers
Electricity is demanded by all electric consumers before their response to the market price. Besides the demand at the spot market, there are demands for frequency reserve qualities.
Flexible consumers
The price-elastic part of the demand is provided by demand-side response (DSR) consumers. DSR consumers can be for example the following.
- Electric vehicles
- Electric air conditioning
- Electric storage heaters
- Electric heat pumps
- Electrolyzers and methanation facilities
- Other flexible consumption like aluminum, paper, steel and cement production processes
Following image depicts the DSR load-adjustment behaviour. Loads can be adjusted in different types: unidirectionally (increase or decrease), bidirectionally (twoway) or as shifts in time (shift). All load adjustments can only occur within the range of the upside and downside potential for each interval. In case of shifts, the sum of single forward-shifts and backward-shifts are bound by the load-increase and load-decrease potential for each interval. Thus, the upside and downside potentials cannot be exceeded in any type. Further, shifts can only occur within a predefined time-shift span (maximum time shift potential).

DSR consumners are active at the spot and frequency reserve markets. Fundamentally, the DSR dispatch follows price incentives. Shifters will have for example a load-decrease at higher prices and the counter load-increase at lower prices. Non-shifters like load-decrease potentials will be used as soon as the electricity price lies above their activation price.
Renewable energy sources
The feed-in of renewable energy sources (RES) can be originating for example from following producers.
- Biogas power plants
- Biomass power plants
- Geothermal power plants
- Micro cogeneration units
- Waste incineration power plants
- Run-of-river power plants
- Marine power plants
- Solar panels
- Concentrated solar power systems
- Onshore wind turbines
- Offshore wind turbines
RES power plants are active at the spot and frequency reserve markets. Fundamentally, the RES dispatch follows price incentives. The feed-in will occur as soon as the market price exceeds the variable cost. Some RES power plants are not flexible and cannot change their feed-in.
Battery storages
Battery storage systems are for example following stationary facilities and mobile applications.
- Automotive batteries
- Charging stations/Grid-to-Vehicle (G2V)
- Charging stations/Vehicle-to-Grid (V2G)
- Electric vehicle batteries
- Hybrid power systems with batteries
- Prosumer-scale batteries
- Utility-scale batteries
- Second life batteries
Battery storages are active at the spot and frequency reserve markets. Fundamentally, the battery dispatch follows price incentives. Discharging will occur at higher prices and charging at lower prices.
Hydro power plants
Hydro power plants are example following facilities.
- Pumped-storages
- Hydro power storages
- Hydro power dams
- Hydro power plant networks
Following image depicts the hydro power plant basis topology. Each hydro power plant is a hydro network and consists at least of one upper reservoir, one lower reservoir and one turbine or one pump. A reservoir can be an dam, lake or constructed reservoir.
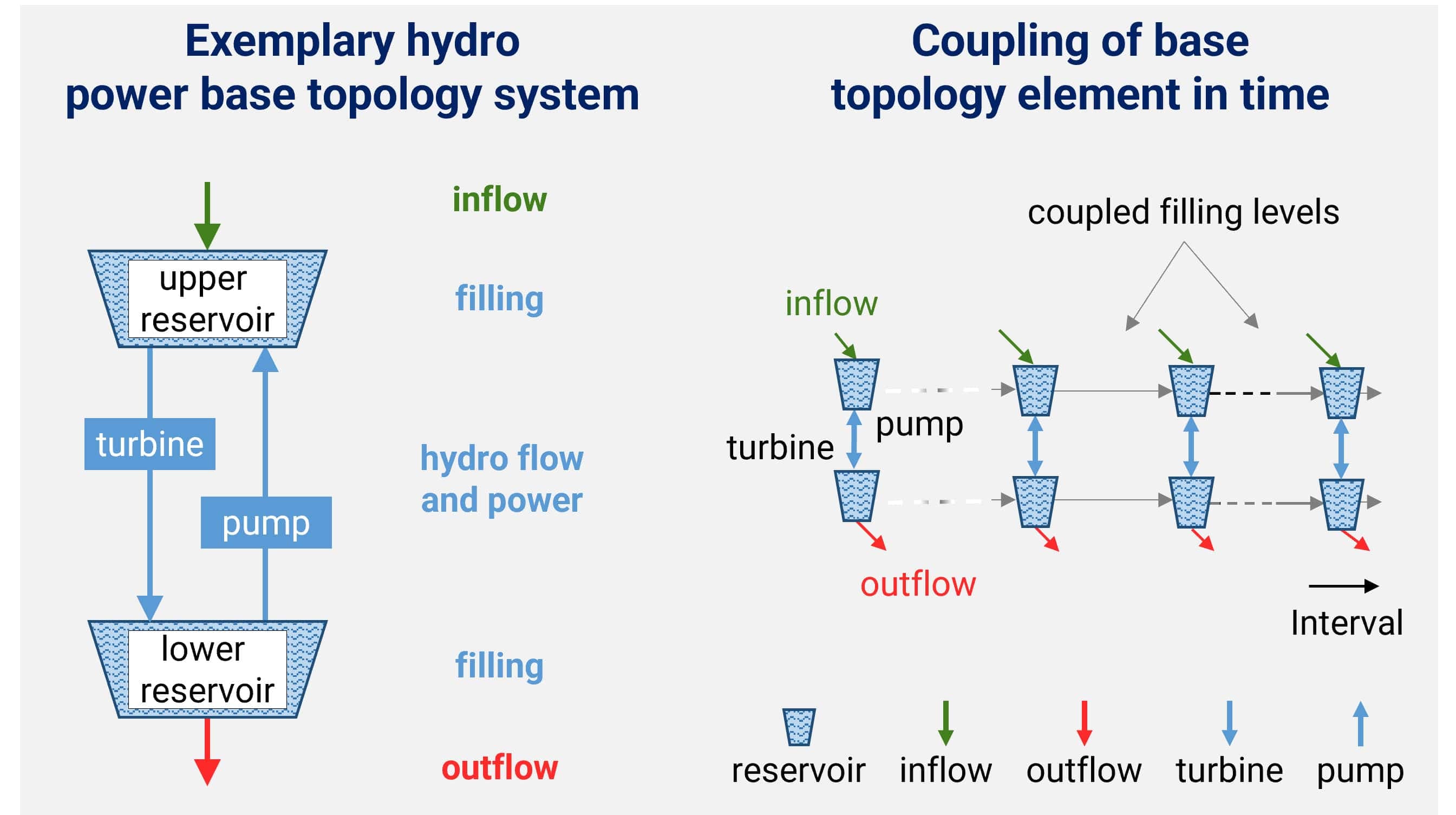
Hydro networks have hydro flows through hydro turbines and pumps between reservoirs. Hydro power cascades link a series of reservoirs with turbines. Turbines, pumps and reservoirs are static as well as inflows that can occur through precipitation and snow melt. The dispatch is bounded by diverse technical and operational restrictions fulfilling the individual characteristics of turbines, pumps, and reservoirs. Hydro flows are linked in turbines to electricity generation and in pumps to electricity consumption. Hydro reservoirs lying above lower reservoirs enable to store the water with the potential energy based on the fall of height.
Hydro power plants are active at the spot and frequency reserve markets. Fundamentally, the hydro power dispatch follows price incentives. Turbining will occur at higher prices and pumping at lower prices.
Thermal power plants
Thermal power plants can be fueled for example with following energy sources.
Following image depicts the influences on the thermal power plant dispatch. Each thermal power plant consists at least of one component.
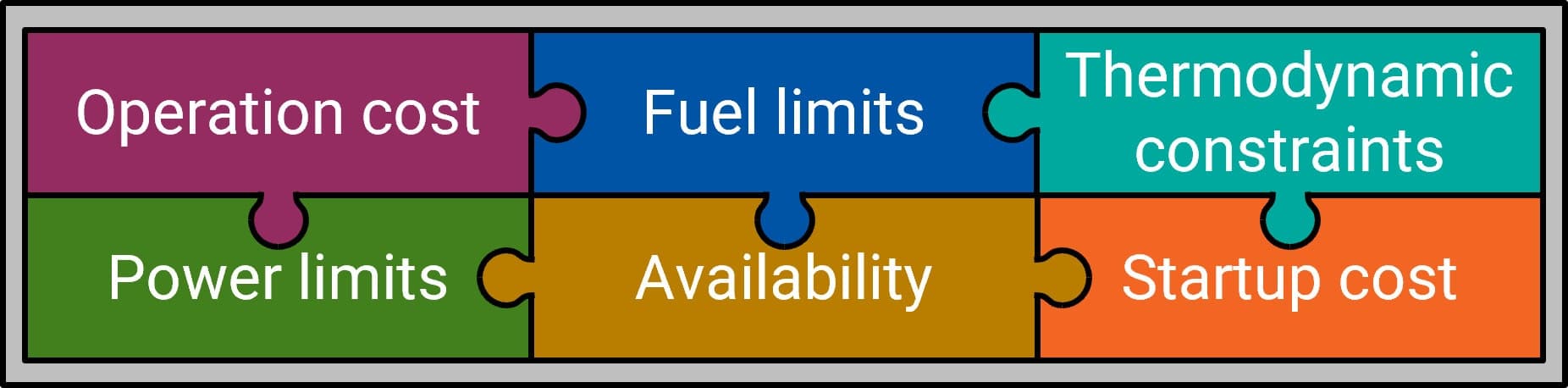
Thermal power plants are active at the spot and frequency reserve markets. Fundamentally, the thermal power plant dispatch follows price incentives. They are generating, as soon the market revenues cover their variable generation cost.
Interconnectors
Following image depicts the exchange capacity types that define possible imports and exports between bidding zones.
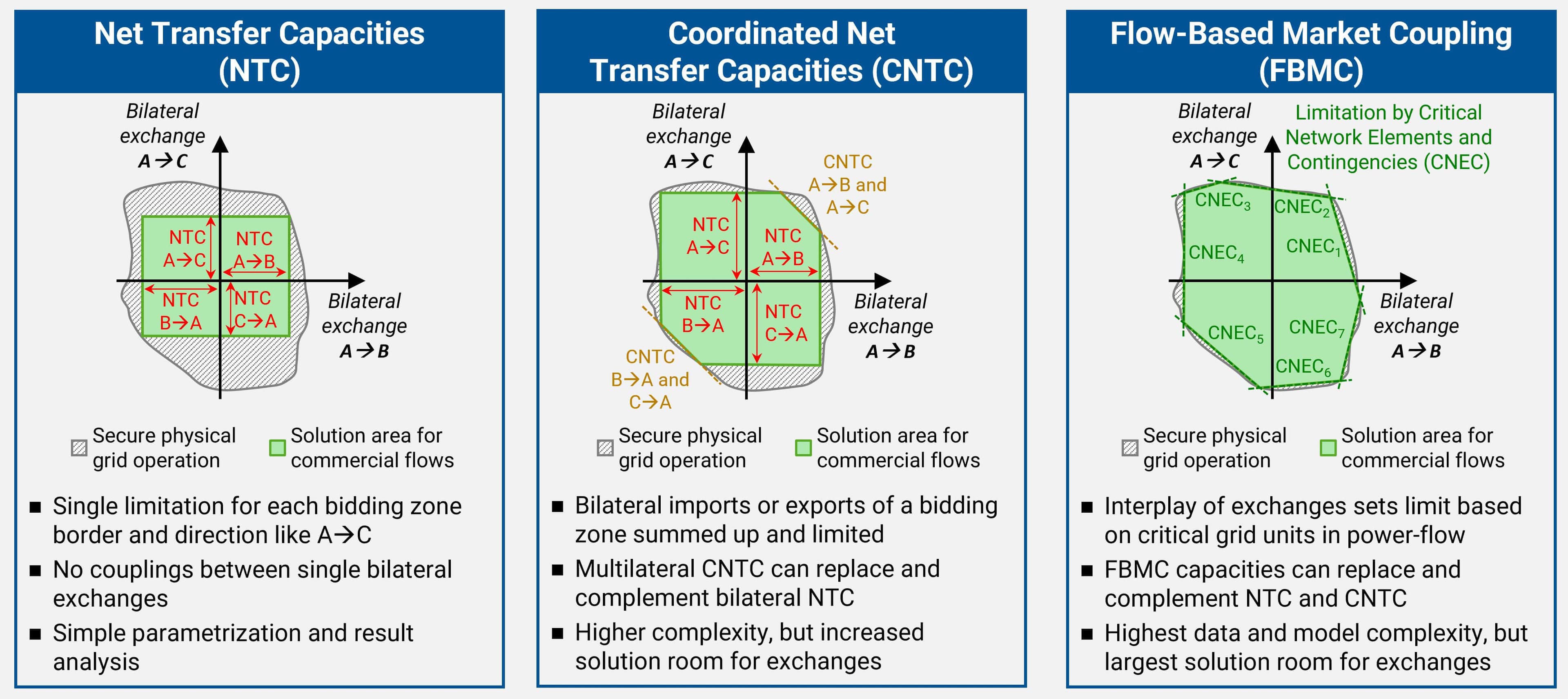
Restrictions formed by critical network elements and contingencies (CNEC) in the flow-based market coupling (FBMC), coordinated net transfer capacities (CNTC) and net transfer capacities (NTC) can occur at the same time. Additionally, reserve exchanges can occur.
The exchanges maximize the social welfare at the electricity wholesale markets. The commercial power flows go usually from the bidding zone with the lower price to the bidding zone with the higher price.
Dispatch vs. Investment
The supply and demand for electricity determine the market equilibrium, which is reflected in market clearing prices and volumes. These short-term dispatch decisions are influenced by long-term investment choices regarding facilities and their capacities. Investments in facilities that will participate in the wholesale market require significant financial resources, so these decisions are made with careful consideration of economic viability assessments (EVA).
The EVA relies on projected future operational revenues and costs, which primarily depend on anticipated short-term dispatch and electricity prices. Forecasted future cash flows, including revenues, operational costs, and investment costs for an investment option, are analyzed using metrics such as net present values (NPV), equivalent annual cost (EAC), and internal rate of return (IRR). These metrics are essential for conducting the EVA.
The cumulative effect of all investment decisions shapes future capacities in the long run, thus defining the long-term market equilibrium.
Units
The electricity market is based on power and work amounts, making definitions and units essential for its understanding. Energy can be defined with following SI units:
- Joule (J): Joule is the unit of energy that equals one watt-second (Ws).
- Watt-hour (Wh): Hatt-hour (Wh) is a unit of energy that is commonly used in household meters in the form of kilowatt-hours (kWh) and in electricity wholesale markets in megawatt-hours (MWh). One watt-hour (Wh) equals the amount of electrical energy expended by a one-watt load (W) drawing power for one hour (h).
Both, joules (J) and watt-hours (Wh) are commonly used units for measuring electric energy. For example, a computer consumes power (measured in watts) over a specific duration (measured in seconds), resulting in a certain amount of energy used. The watt is a unit of energy per time, while the hour is a unit for measuring time. When watts are multiplied by hours, the resulting unit of energy is called the watt-hour. This represents the amount of power (in watts) consumed over a given number of hours.
In the context of the electricity wholesale market, the following table presents important constants.
Number | Description | Importance |
---|---|---|
8760 | Hours of non-leap calendar year | Years are used by default as scenario time ranges so it allows to check if a series of hourly values represents a complete year or has data missing |
8784 | Hours of leap calendar year | Leap years can be used as scenario time ranges so it allows to check if a series of hourly values represents a complete leap year or has data missing |
168 | Hours of calendar week | Weeks represent one main load-cycle |
3.6 | Factor to convert MWh to GJ with 100 % efficiency | 1 megawatt-hour equals 3.6 gigajoules so that this conversion allows to compare and check fuel prices (in EUR/GJ) and electricity prices (in EUR/MWh) |